Definition of wood
Wood is a renewable natural resource found in the roots, trunks, branches and twigs of trees and shrubs.
This fascinating material plays a crucial role in numerous aspects of our lives, whether as a building material for structures and furniture or as a valuable source of energy.
In chemical terms, wood consists mainly of carbon (48-50%), oxygen (43-45%), hydrogen (5-6%), and small amounts of nitrogen (0.01-0.04%) and ash (0.6%).
The major constituents of wood are cellulose, hemicellulose and lignin, while extractives and ash are minor constituents. The unique diversity of wood species results from differences in the distribution of these major and minor constituents.
Wood, of course, also proves to be an outstanding material for the production of cutting boards.
Formation of wood
The development of wood begins with a tiny seed that grows into trees. These seeds germinate in the soil, where they absorb water and nutrients. When the seedling sprouts from the soil and develops the first leaves, the process of differentiation of the various cells and tissues is complete and the process of photosynthesis comes into play.
In this process, plants (and certain bacteria) use light energy and chlorophyll (in the chloroplasts) as a catalyst to convert water and carbon dioxide into energy-rich glucose (dextrose) and, with the subsequent elimination of oxygen, starch is formed from glycose.
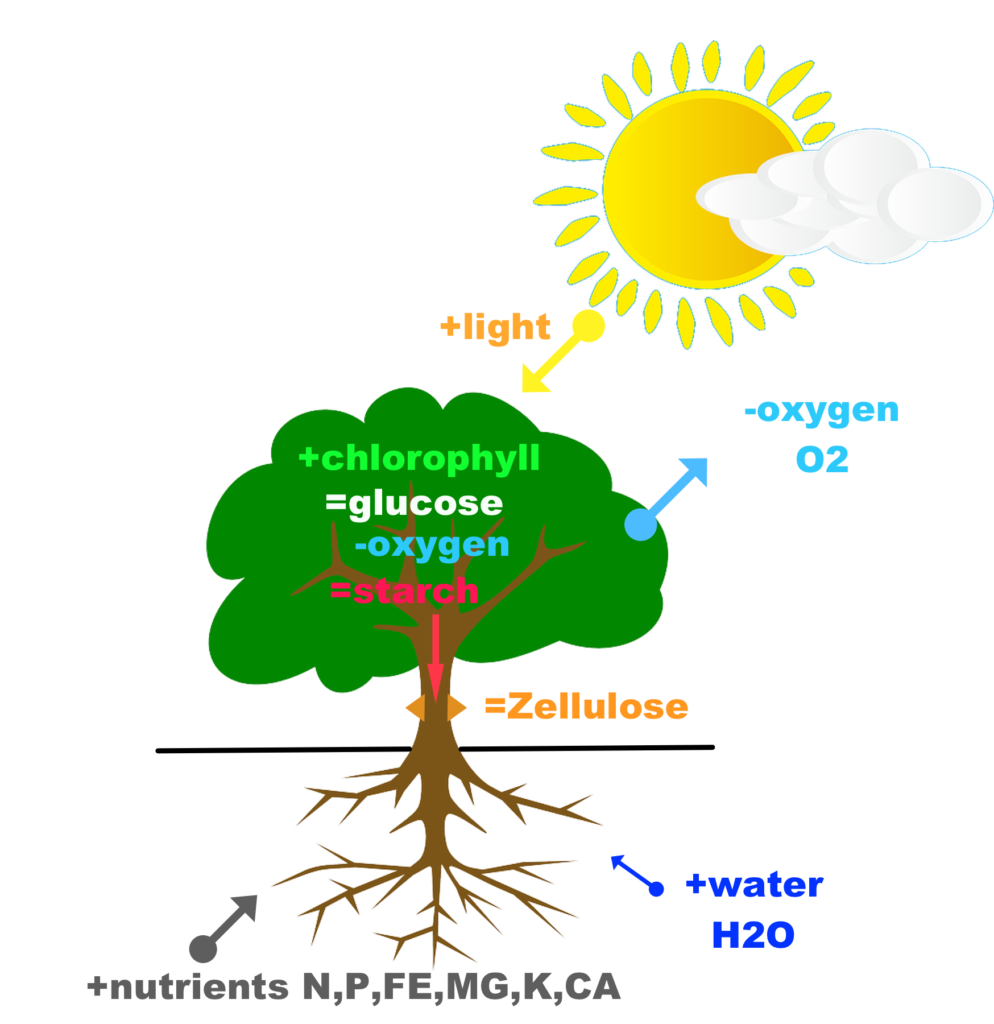
The resulting starch forms the basic building block of cellulose, while the oxygen that is split off (assimilation) is a by-product of photosynthesis.
Carbon dioxide enters the leaves through tiny openings (stomata, pallisade cells) on the underside of the leaves, while nutrient-enriched water is transported to the leaves by osmosis through the fine root hairs and sapwood (xylem). The absorbed water from the roots contains various nutrients such as nitrogen, potassium, phosphorus, iron, magnesium, calcium and trace elements.
The starch-containing sap from the leaves is passed down the bast tissue (phloem) and used in the adjacent cambium to form wood and bast cells for further growth of the tree.
The transport of nutrients and water from the roots to the leaves takes place through the sapwood (xylem), while the starchy plant sap flows from the leaves towards the roots through the bast tissue (phloem).
The actual formation of wood takes place in the so-called cambium, which is located between the bast (phloem) and the sapwood (xylem). Cell divisions occur here, producing wood cells on the inside (sapwood area) and bast cells on the outside.
As the tree grows, the sapwood area expands during the seasonal growth phases and the trunk becomes thicker. During the winter or dry seasons, the tree slows its growth and produces less sapwood. This can be seen from the annual rings (growth rings).
In the outer sapwood area, nutrient-rich water is transported to the leaves, and at the same time the tree stores nutrients in it, in the parenchyma cells (storage tissue), which are visible as pith/wood rays in cross-section.
The inner sapwood area hardens due to chemical changes and forms the heartwood, which can take on a different structure and color. This explains the different hardness and sometimes different color between the outer sapwood and the inner heartwood.
The role of wood in nature
Wood plays an essential role in nature by performing several important functions. One of these functions is to provide stability to trees and allow them to reach impressive heights. Even in strong gusts of wind, they can withstand due to the strength of the wood.
In natural ecosystems, wood plays a crucial role in the habitat of numerous plant and animal species. It serves as a shelter for animals such as birds and insects and becomes a food source for fungi as well as other organisms involved in the decomposition process of dead wood. The forest performs other important functions, including storing water and carbon, protecting against wind, controlling soil erosion, filtering dust, and regulating humidity.
In addition, wood acts as a natural carbon dioxide store. Trees are among the largest producers of oxygen by absorbing carbon dioxide from the atmosphere through photosynthesis and converting it into energy-rich glucose. Glucose contains carbon, which is stored in the wood.
Over the course of a year, a tree absorbs an average of about 25 kilograms of COâ‚‚, which is equivalent to about 2 tons over 80 years. This carbon dioxide is stored in the form of carbon in the tree trunk. When wood is burned, the carbon is converted back to carbon dioxide and released. Overall, wood fulfills a diverse and indispensable role in the ecosystem.
Wood species
In botanical classification, woods are divided into hardwoods and softwoods, and are referred to as “hardwoods” and “softwoods” in English. It is important to note, however, that this classification has primarily nothing to do with the hardness of the wood, as I have this article described in detail in this article.
Hardwoods (hardwoods)
Deciduous trees belong to the group of angiosperms, that is, to the angiospermous plants that produce flowers in which the seeds are located in the ovary. These angiosperms can be divided into two groups based on the number of cotyledons in their seedlings: Monocotyledons(monocotyledonouslike bamboos and palms) and dicotyledons(dicotyledonous like oaks and elms).
A deciduous tree is divided into:
The crown of a tree performs the essential task of metabolism, especially photosynthesis, and plays a crucial role in the formation of fruit.
The trunk is the supporting element, carries the crown and serves for the production of wood.
The root anchors the tree firmly in the ground and allows it to absorb water and nutrients through its fine root hairs.
Deciduous trees are divided into several categories depending on whether they bear fruit and what kind. These include fruit trees (pear and apple trees), nut trees (walnut trees) and deciduous trees that do not bear edible fruit (linden).
A further distinction of deciduous trees is made into two main categories: Deciduous trees, which shed their leaves seasonally, and evergreen trees, which retain their leaves throughout the year.
Deciduous trees, which comprise the majority of deciduous tree species in temperate climates, are characterized by the seasonal shedding of their foliage. In contrast, deciduous evergreen trees, which are mainly found in subtropical and tropical zones, retain their leaves throughout the year.
Compared to conifers, deciduous trees tend to have a more limited geographic distribution because they are less common in northern latitudes. Nevertheless, they have an impressive biodiversity.
Deciduous trees tend to grow more slowly, which increases their degree of hardness compared to softwood in most cases. The hardwood is usually traded more expensive than softwood. Hardwood processing plays an essential role in interior finishing and furniture production. Due to their higher dead weight and not always so uniform growth, they are less used in engineering timber construction.
Due to the degree of hardness of hardwood, it is generally more suitable for the manufacture of cutting boards.
The cell structure of hardwoods is more complex than that of softwoods.
Softwoods
Conifers, also known as conifers, are among the oldest trees in evolution and have a comparatively less differentiated cell structure than hardwoods.
These trees belong to the group of gymnosperms because their ovules are exposed and not surrounded by an ovary.
The leaves of conifers are typically needle-shaped or scale-like and usually retain their green color throughout the year, with exceptions such as larch.
Conifers are found in a wide range of climates from cold to temperate regions, and occasionally in the rainforests of the Southern Hemisphere. They dominate the tree population in many northern regions.
In most cases, conifers are characterized by fast growth and tend to be softer, with some exceptions such as yew.
Due to their lower dead weight and more uniform structure, softwoods are often used in timber construction.
For the manufacture of cutting boards they are rather less suitable.
Chemical composition of wood
As already mentioned, wood is composed of the following components in addition to the water stored in the cells:
Main components
- Cellulose (40% to 60%)
- Hemicellulose (6% to 27%)
- Lignin (18% to 41%)
Ancillary components
- Extractives (2% to 7%)
- Ash (0.2% to 0.6%)
Main components
Long-fiber polysaccharides, namely cellulose and hemicellulose, are found in the cell walls of wood.
Cellulose is the dominant component and gives the wood its tensile strength. It has a relatively high chemical resistance. Hemicellulose forms the remaining polysaccharides in the cell wall. Cellulose is light in color.
Lignin is a biopolymer that is difficult to biodegrade. It is the second largest component of the wood cell wall after cellulose and is formed at the end of cell growth. It is extremely strong, brittle, water repellent and brown-reddish in color. Together with hemicellulose, lignin contributes to the lignification of the cells and the compressive strength of the wood.
In addition, lignin protects the cellulose from moisture and degradation by microorganisms by cementing the cell wall components together. This gives the wood its longevity, even after processing. In addition, lignin serves as protection against insects and fungi.
Wood that is kept permanently dry or continuously under water has an almost unlimited shelf life.
Decomposition of lignin by fungi results in white rot, while in brown rot the cellulose is broken down, leaving the lignin in a brown form. The lignin content is often higher in softwoods than in hardwoods, which makes them more resistant to chemicals, among other things.
The different distribution of cellulose, hemicellulose and lignin in different types of wood leads to diverse properties and applications of wood. It is the interaction of these main components that makes wood resilient to tension, bending and compression, which enables trees to grow to height.
Ancillary components
The distribution of organic extractives, including resins, waxes, fats, oils, tannins, and dyes, and the presence of inorganic ash, which includes magnesium, phosphorus, potassium, sodium, silica, silicates, phosphates, chlorides, sulfates, and metals, are other factors that affect the chemical and mechanical properties of wood. This distribution and composition vary by wood species and contribute to wood species specificity.
A characteristic scent, such as the smell of freshly cut coniferous wood, is due to the presence of the extractive resin in the tree.
The structure of wood
The cell structure of hardwood and softwood differs in several aspects, which justifies the separate consideration of the two types of wood.
Coniferous wood cell structure
The cell structure of softwood differs significantly from that of hardwood.
It is less complex overall and is characterized by two basic cell types: Tracheids and parenchyma cells, also known as storage or medullary rays.
The tracheids are the main components of coniferous wood. These elongated fiber cells run parallel to the trunk axis and taper at the ends. In contrast to hardwood, the tracheids in coniferous wood perform a dual function: they not only provide stability, but also transport water.
Earlywood tracheids, which are thin-walled and wide-lumened, are responsible for vertical sap transport, while latewood tracheids, which are thick-walled and small-lumened, provide conifer strength.
The transport of water and nutrients in earlywood tracheids occurs via so-called yard stipples. These small cross-connections in the cell walls between neighboring cells act as a kind of valves and regulate the flow of fluid between the leading cells.
The structure and surface quality of the coniferous wood are largely dependent on the size and diameter of the tracheids.
Parenchyma cells, which function as storage cells, make up only about 5% of the cell tissue. They are divided into strand parenchyma cells, which are axially oriented and parallel to the stem axis, and ray parenchyma cells, which run horizontally, as wood rays, toward the stem center.
Unlike hardwood, softwood does not have conductive tissue and is therefore referred to as non-porous.
However, some softwoods, such as pine, spruce, larch, and Douglas fir, have resin channels, also known as epithelial cells, that run both vertically and horizontally.
The resin produced, which can heal injuries, is transported through these channels to the affected parts of the tree. Resin production is triggered by bark injury or pest infestation.
Hardwood cell structure
As already indicated, the cell structure of hardwood is much more diverse and complex compared to softwood.
In hardwoods, we find a broader range of cell types, including tracheids (vessels or guide cells), sclerenchyma cells (fiber cells) for support, and parenchyma cells (visible as wood/marrow rays) that function as storage cells.
In contrast to softwoods, where the tracheids perform both strengthening and water-conducting functions, in hardwoods there is a clear separation of these tasks.
Water and nutrients are transported in hardwoods by tracheae (vessels or pores), which consist of thin-walled, wide-lumened, tubular, contiguous cells that are larger in diameter.
The strengthening tissue is located between the leading tissue and consists of smaller wood fiber cells with solid cell walls, which can be divided into libriform fibers and fiber tracheids.
The storage tissue consists of parenchyma cells, which are divided into longitudinal parenchyma (axial) and ray parenchyma (radial, wood rays). These cells store nutrients in radial and axial direction. In some species, such as oak, the wood rays can be particularly pronounced.
In contrast to softwoods, hardwoods have pores and are therefore also referred to as“porous wood species“. The pores visible in cross-section are the severed tracheae or vessels.
Depending on the arrangement, size and quantity of the pores, they are classified as ring-porous, semi-ring-porous or dispersed-porous.
Ring-porous wood species such as oak and ash have unevenly distributed pores. Beech, pear and maple, on the other hand, have an even distribution of pores and belong to the dispersed-porosity wood species.
American walnut and cherry belong to the semi ring-porous species, a transitional form between ring-porous and scattered-porous. Their pores are relatively evenly distributed over each growth ring, but show marked differences in size.
A further classification into fine-pored (maple, birch, beech, ash or cherry) and coarse-pored wood (oak, chestnut, walnut, teak) is based on the diameter of the vessels. Coarse-pored wood has larger and clearly visible pores, while fine-pored wood has smaller and less visible pores.
The attached figure illustrates the relative speed of water transport in the sapwood region of trees. Measurements were made at noon at a height of 1.30 meters, and significant differences are evident in terms of porosity and transport velocity.
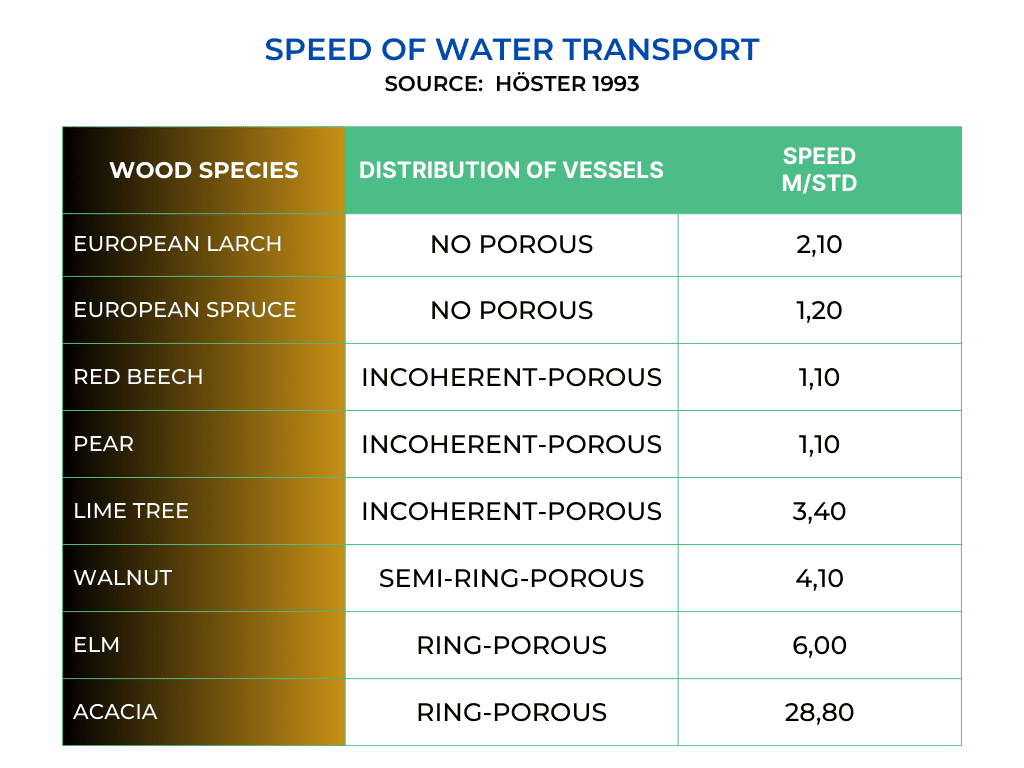
Wood in longitudinal and cross section
Longitudinal section
To make the best use of wood, there are several methods of cutting a log lengthwise(parallel to the log axis). These longitudinal sections essentially comprise the radial section and the tangential section.
In the radial cut (also called mirror cut ), the cut is parallel to the trunk axis and runs near the center of the trunk. This results in the annual rings appearing slightly inclined to nearly upright and parallel. The medullary rays are usually bright and often shiny (mirror-like), and they always end at the bast tissue.
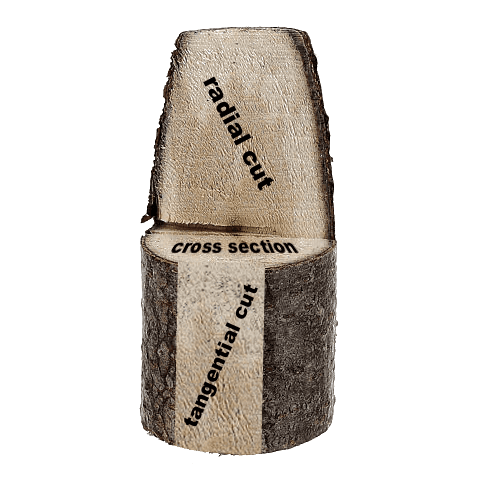
The longitudinally split large vessels are called needle cracks. The expression of these cracks varies depending on the type of wood; in oak, ash or walnut they are more coarse-pored, while in beech or maple they are small-pored.
Wood that has been cut radially is less prone to warping or bending, and it allows a clear representation of the different zones in the tree. For this reason, the radial section is particularly important for determining the type of wood.
In tangential cutting (also called chordal cutting or flap cutting ), the cut is made parallel to the trunk axis, but outside the center of the trunk. The annual rings are cut tangentially to horizontally.
The resulting boards are more prone to warping, but offer the visually appealing conical grain of the wood (veining). In this cut, the medullary rays are cut at right angles.
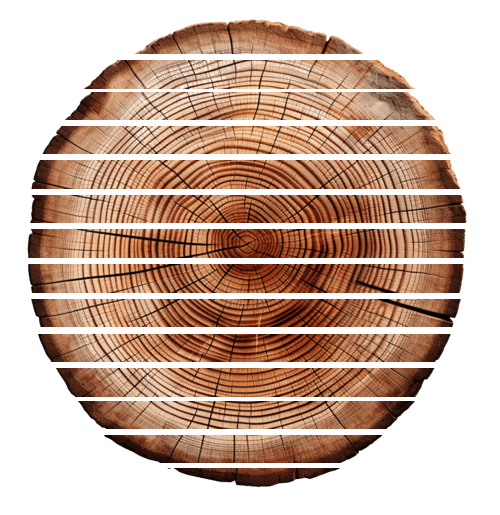
Round cut
This is the most common variant. This cut gives boards with tangential to standing annual rings, as well as the medullary tissue. The amount of waste is small.
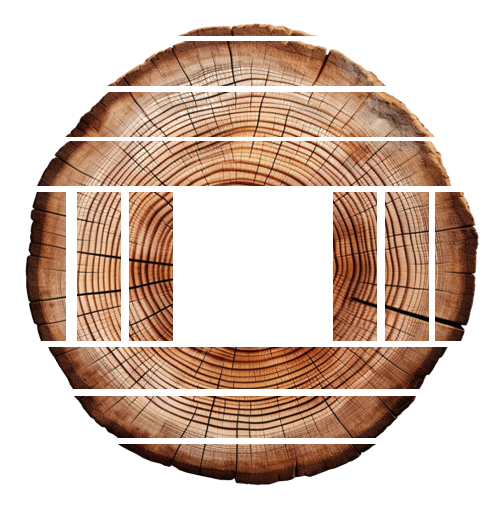
Simple cut
In this process, only horizontal annual rings are produced, without pith. Here, too, the waste is small.
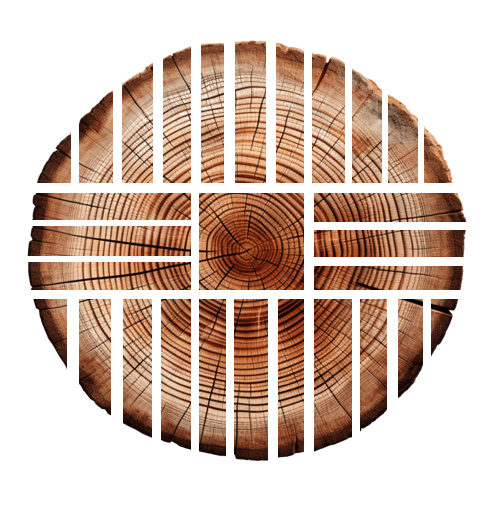
Traditional rift cut
In rift cut, the boards consist almost entirely of standing annual rings, without pith. The waste is greater, but the resulting boards are particularly stable in shape.
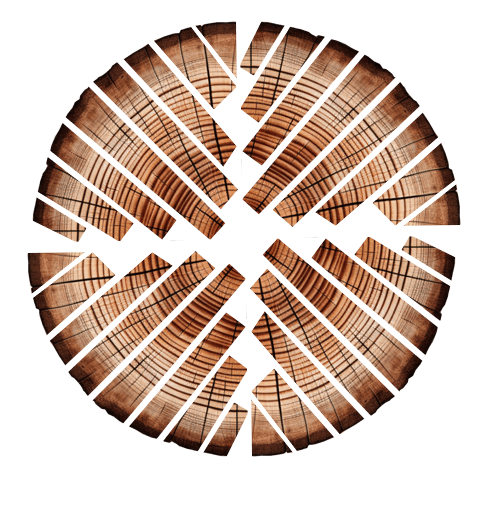
Edelrift Cut
Similar to the rift cut, but with an even higher blend. Here, too, the boards consist almost exclusively of standing annual rings and without pith. The peculiarity lies in the extraordinary dimensional stability of the boards.
These different cutting options offer flexibility in wood processing and meet different requirements depending on the desired wood quality and intended use.
Cross section
Tissue types in cross section
The cross-section of a log – also called end grain – is perpendicular to the log axis and best shows the different tissue types of the wood.
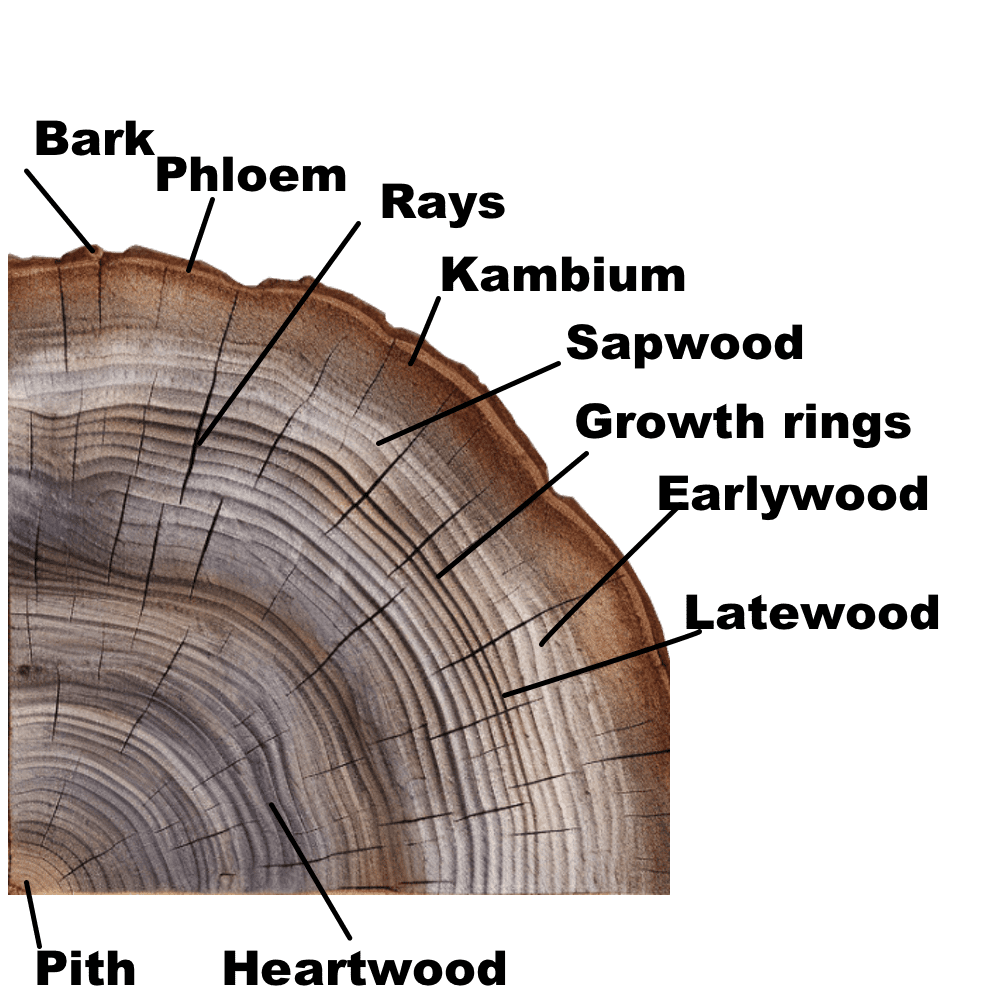
The pith (also called the pith) represents the tissue in the axis of the tree trunk and is located within the first growth ring.
In older trunks, it usually has a diameter of a few millimeters and plays a crucial role in the longitudinal growth of the tree. It is clearly distinguished from the surrounding wood by its darker color and structure. When processing wood, the pith can become either completely or partially visible in the radial cut and contrast in color. This feature is a characteristic part of the natural appearance of wood.
The core of the tree is an integral part of the trunk and serves to stabilize it. Heartwood is characterized by its increased hardness, dryness and usually darker color. These characteristics result from chemical changes in the cells of the inner sapwood.
Mature wood – Some tree species exhibit what is known as mature wood, which is found between the sapwood and heartwood areas. This is the part of the sapwood that can turn into heartwood in parallel and darken over time. In tree species such as spruce, copper beech, linden and pear, this transformation does not occur and their heartwood resembles the color of their sapwood, but is drier and harder.
The sapwood (xylem) is adjacent to the heartwood zone and consists of cells that are still partially alive. This wood transports water as well as nutrients from the root to the crown. The sapwood is often lighter in color and softer than the heartwood. It also contributes to stabilization and, in addition, the nutrients produced by the leaves are stored in it. In sapwood trees such as poplar, white beech or sycamore maple, the entire trunk cross-section consists of sapwood.
The cambium is a very thin cell division tissue (also called formation tissue) that lies between the sapwood area and the bast. Here the new wood is formed by cell division. New wood cells are formed on the inside of the cambium and new bast cells on the outside. In the cambium, the growth of the stem takes place in the width.
The bast (phloem) is the inner living part of the bark. This is where the nutrient-rich plant sap (products of photosynthesis) is channeled from the leaves or needles to all the growth zones of the tree. It forms the lifeline of the tree.
The bark is the outer dead part of the bark. Due to the continuous thickness growth of the wood, the bark cracks more or less depending on the type of wood. The dead layers then form the bark, which serves as a protective and insulating layer against injury, weather and climate. Bast and bark together form the bark.
Annual rings (earlywood and latewood)
A growth ring, also called a growth ring, is composed of the layers of earlywood and latewood. The boundaries between annual rings are created by the distinctive transition from englumed, darker latewood to wide-lumed, lighter earlywood.
During the growing season in spring, the cambium produces wide-lumen cells toward the sapwood. These cells are large-pored and thin-walled, which makes them ideal for transporting nutrient-rich plant sap from the roots. The early wood, the lighter part of a growth ring, has a less dense structure and is mainly responsible for nutrient transport.
The dormant period gives rise to the latewood, which consists of englumed, smaller cells with thicker cell walls. This makes it stronger than the early wood. The resting periods in the annual ring are usually narrower and darker than the growth periods. The late wood mainly contributes to the strength of the wood.
The annual rings in a cross-section of a tree trunk are characteristic of trees growing in climates with distinct seasons. In contrast, annual rings are not found in areas without clear seasons, such as subtropical zones.
The annual rings provide information about the age and growth of the tree. For example, closer annual rings provide information about slow-growing tree species (boxwood), location (open terrain, higher elevation), environmental conditions (low soil moisture, drought), or short vegetation period (northern latitude).
Annual rings do not have to be uniformly thick and concentric in cross-section. The prevailing wind direction, slope of the terrain as well as diseases and fire impact can cause an uneven arrangement of the annual rings.
General properties of wood
Mechanical-physical characteristics
Bulk density
The bulk density of a wood describes the ratio between its mass and its volume and is usually expressed in kg/m³ or kg/dm³. This value is strongly dependent on the wood moisture content, which is usually still very high in freshly cut wood. In this state, the wood is heavier and softer compared to its dried form, as illustrated in the following example:
Oak per cubic meter weighs 1.000kg when wet, but only 670kg when dried.
In order to obtain comparable values, the gross density is usually given at 12% wood moisture content, which corresponds to long-term storage in a normal climate at 20°C and 65% relative humidity.
The bulk density depends on the wood structure at the same drying state.
Without including the cell cavities, the density of the pure cell wall substances is about 1,500kg/m³!
It is only due to the wood species-specific cell structure with the cavities and intercalations that the bulk density varies, as some wood species have many cavities, others only a few.
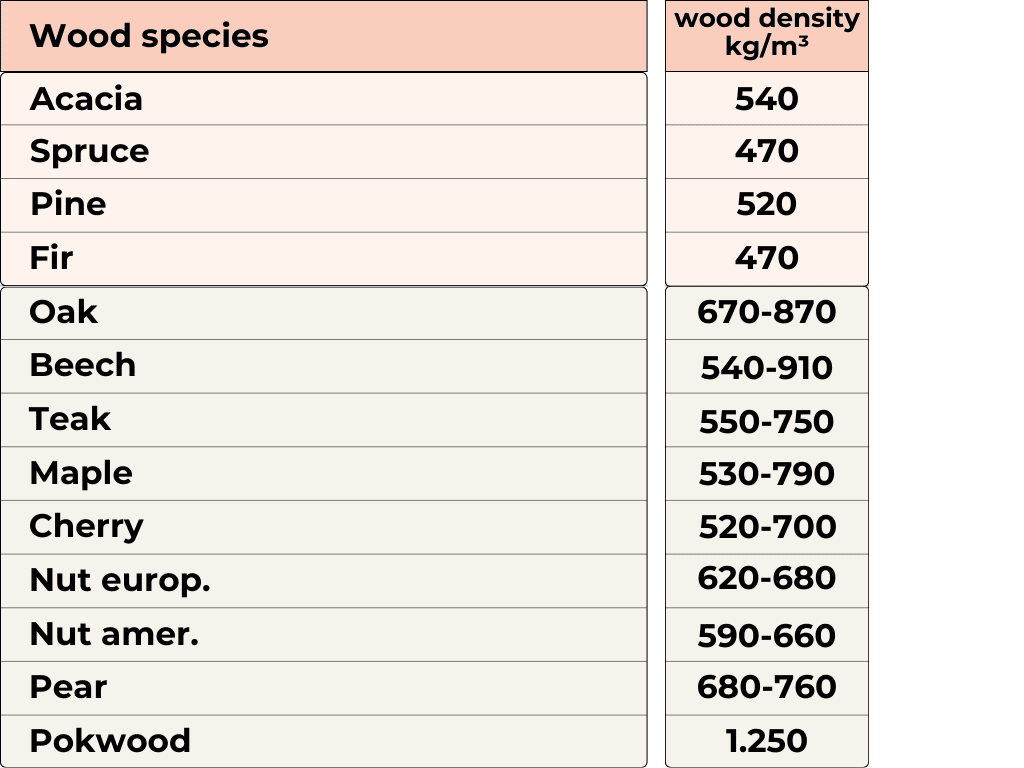
Softwoods in particular have a remarkable number of cavities, which is why they are usually lighter than hardwoods. In contrast, the cell walls show hardly any differences between the different wood species.
As the bulk density increases, the cell voids decrease, resulting in an increase in mass, elastomechanical properties, hardness, strength and thermal conductivity. At the same time, the shrinkage and swelling dimensions decrease.
Wood hardness
Wood hardness refers to the resistance of wood to penetration by a body (tool) and is one of the most important properties, especially when choosing wood for a cutting board. Wood hardness is divided into Janka and Brinell hardness expressed. It is influenced by the following factors:
Bulk density
Je höher die Rohdichte des Holzes, desto härter ist es in der Regel. Dies liegt daran, dass Holz mit hoher Rohdichte mehr festere Zellwandsubstanz und weniger Hohlräume enthält, was zu einer höheren Festigkeit führt.
Moisture content
Generell gilt, je höher der Holzfeuchtegrad, desto weicher wird das Holz im Vergleich zu trockenerem Holz. Dieser Effekt ist auf die Lockerung der Wasserstoffbrückenbindungen zwischen den einzelnen Zellulosemolekülen zurückzuführen.
Growth conditions
Bäume, die unter ungünstigen Bedingungen wachsen, produzieren oft härteres Holz, um sich besser vor äußeren Einflüssen zu schützen.
Age
In der Regel wird das Holz älterer Bäume aufgrund einer höheren Rohdichte und einer längeren Einlagerung von Zellwandsubstanz (Verholzung) härter.
Wood fabric type
Splintholzbereiche sind weicher als das Kernholz. Spätholzbereiche innerhalb der Jahresringe sind härter als die zugehörigen Frühholzbereiche. Bereiche unter Ästen sind durch die ständige Belastungen härter.
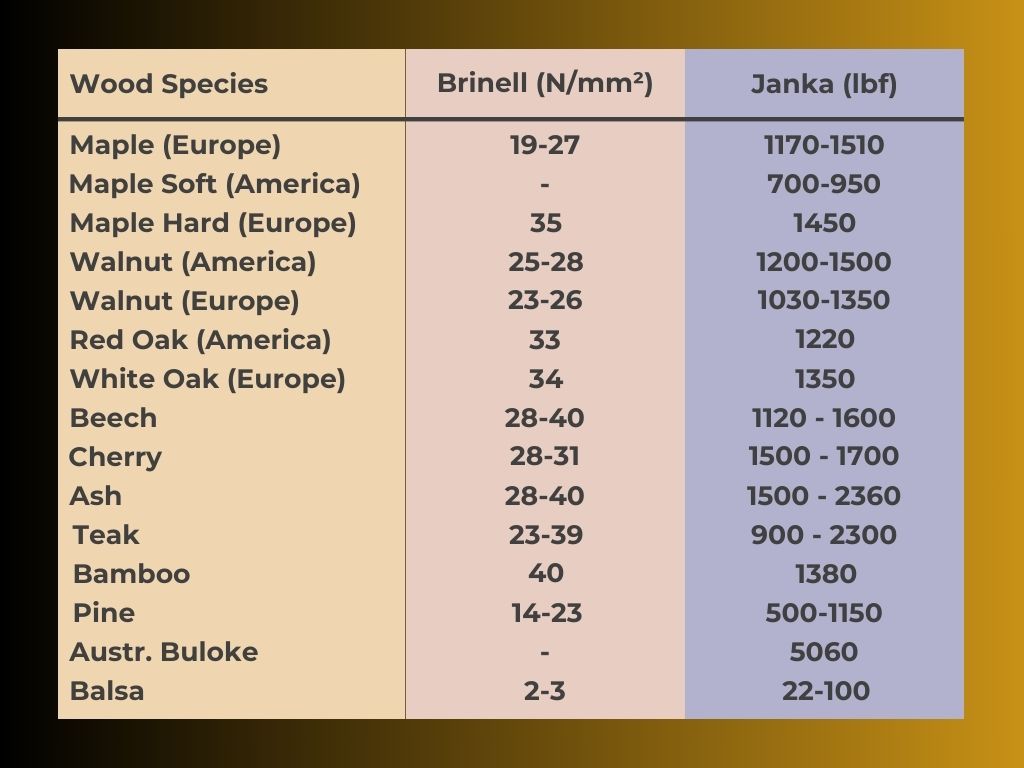
Wood strength
Wood is an anisotropic material, which means that it has different strengths in different directions. In principle, wood is more resilient in the direction of the grain than across it. Values are determined at 12% wood moisture content and given in N/mm². Depending on the angle of the applied force to the direction of the grain, the following types of loading are distinguished for wood:
- Compressive loads occur when a force perpendicular to the fiber axis acts on the wood (support).
- Tensile loads occur when a tensile force acts on the wood in the direction of the grain.
- Bending loads occur when a force acts on the wood transverse to the fiber axis (beam).
- Shear stresses occur when a force parallel to the fiber axis acts on the wood.
- Torsional loads occur when a force is rotated about the fiber axis.
The differences can be seen very clearly in the example of oak:
Fracture strength in tension 110 N/mm², in compression 52 N/mm², in bending 95 N/mm² and in shear 11.5 N/mm².
In general, the strength of wood increases with increasing gross density and a higher proportion of latewood (heartwood). At the same time, higher moisture content and higher temperatures lead to a reduction in wood strength.
Wood species such as beech, oak and walnut are characterized by particularly high strength. In contrast, most conifers and fast-growing hardwoods such as poplar belong to the less solid wood species.
Thermal conductivity
The thermal conductivity of wood refers to its ability to conduct heat. In the direction of the wood fiber, this conductivity is about twice as high as perpendicular to the fiber.
Due to its porous structure, wood is basically a poor conductor of heat and therefore shows excellent thermal insulation properties.
This phenomenon is due to the tiny air-filled pores inside the wood. In fact, wood requires about twice the amount of energy to raise its temperature by 1°C compared to concrete. The thermal property of wood is influenced by various factors, including:
- Wood species: Different wood species have different thermal conductivities (spruce: 0.13 W/(mK), beech: 0.1 W/(mK), oak: 0.15 W/(mK)).
- Wood moisture: The thermal conductivity of wood increases with increasing wood moisture. Damp wood conducts heat better than dry wood.
- Bulk density: The higher the bulk density of the wood, the higher the thermal conductivity. Wood with higher bulk density contains fewer voids and therefore conducts heat better than wood with low bulk density.
Durability
Natural durability of wood describes the ability of wood to resist wood-destroying organisms such as fungi, insects and termites.
The classification of natural durability to fungi is based on the following classes:
- Class 1: very durable wood
- Class 2: durable wood
- Class 3: moderately durable wood
- Class 4: little durable wood
- Class 5: non-durable wood
The classification of natural durability to termites is based on the following classes:
- Class D: very durable wood
- Class M: moderately durable wood
- Class S: susceptible wood
The classification of natural durability of dry wood against larvae of beetles is made according to the following classes:
- Class D: durable wood
- Class S: susceptible wood
- Class SH: susceptible also in heartwood
Wood with higher durability is usually more resistant for outdoor use. Here are three examples of wood species:
- Douglas fir: Class 3-4, S
- Oak: Class 2, S
- Beech: Class 5, S
- Pine: Class 3-4, S
- Spruce: Class 4, S,SH
- Larch: Class 3-4, S
Wood with a moisture content of less than 20 percent is resistant to fungal attack.
Humidity properties
The shrinkage and swelling of wood is crucial to its dimensional stability and is often referred to as the“working of wood“. This process varies considerably depending on the type of wood and affects the length, width and height of the wood.
Wood shrinks and swells to very different degrees in its three wood anatomical directions – in the fiber direction(longitudinal), in the wood ray direction (radial) and in the annual ring direction (tangential). The ratio of shrinkage and swelling in these directions is about 1:10:17 (longitudinal : radial : tangential). These differences are due to the arrangement and size of the tissue cells in the wood. Therefore, how the wood was cut from the trunk – radial or tangential – is also important.
The shrinkage and swelling process of wood is a physical process that results from the amount of water that can be absorbed by the cell walls of the wood.
When wood releases water into its environment below its so-called fiber saturation range (this varies depending on the type of wood and is approximately between 25 and 35 percent wood moisture content), it shrinks. Conversely, wood swells when it absorbs water from the environment, also below its fiber saturation range, resulting in a buildup of swelling pressure.
The shrinkage and swelling, especially perpendicular to the grain, varies depending on the wood species. For example, the shrinkage and swelling of oak and larch is lower than that of beech, but higher than that of teak.
Since cutting boards often come into contact with water, how the woods are glued together in terms of fiber orientation is critical in the manufacturing process. Radially cut wood with vertically positioned fibers is preferable.
Sawn timber is divided according to the moisture content of the wood into:
- Fresh sawn timber >30% wood moisture content
- Semi-dry sawn timber 20-30% wood moisture content
- Dry sawn timber <20% wood moisture content
- For cutting boards < 12% wood moisture content
Optical properties
Grain
The characteristic markings and patterns in wood known as grain are created by complex interactions of color, luster, grain pattern, wood fiber arrangement, and a tree’s natural growth habit.
This grain is also influenced by possible anomalies in the tree. Depending on the direction of the cut, different aspects of the grain are revealed, which are visible in the cross section by annual rings, in the tangential section by the grain pattern (veins) and in the radial section by medullary rays. The grain is a crucial factor in identifying the wood species.
Descriptions of the grain range from striped, plain, whimpered, floral, mottled, bubbly, curly, wavy, dotted, pyramidal to swirled or mirrored.
Surface structure
Wood can have a smooth, rough or textured surface, depending on the type of wood and how it is processed. Smooth wood surfaces are created by applications such as sawing, sanding, chiselling or planing. Structured and rough surfaces, on the other hand, are created by flaming, brushing, hewing or chipping.
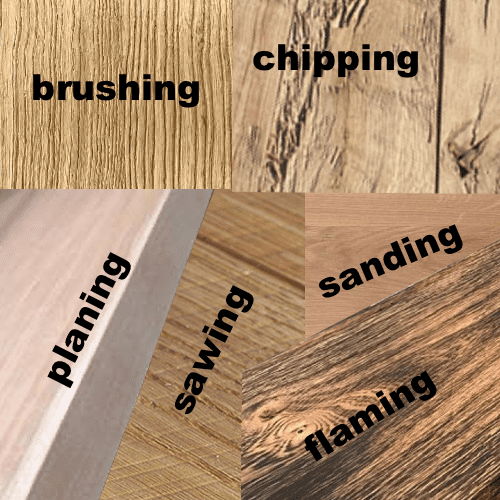
These applications determine the feel of the wood as well as its visual effect in terms of contrast and depth.
Surface plot
The choice of surface treatment, be it the application of oils, waxes, varnishes or glazes, can have a significant impact on the visual characteristics of the wood. These treatments serve not only aesthetic purposes, but also have a protective effect on the wood, see also here.
Conclusion for your cutting board
Knowledge of the many aspects of wood is essential when it comes to creating high-quality cutting boards . In the big picture, wood is the ultimate material for cutting boards, offering a unique symbiosis of functionality, robustness, aesthetics and sustainability.
In this article I have focused on the properties of this valuable material, which are specifically useful for the manufacture of wooden cutting boards. This knowledge will help you avoid mistakes when choosing the right type of wood and perfect the making of a cutting board.
Along the way, you’ll develop a deeper understanding and respect for the fascinating and diverse material that is wood.
Sources: BAUSTOFFKENNTNISS – Scholz/Hiese, WOOD recognize and use – Terry Porter, PRAXISBUCH WOOD – Dorling Kindersley publishing house, wood of the trade – webpage.